Improving Global Health
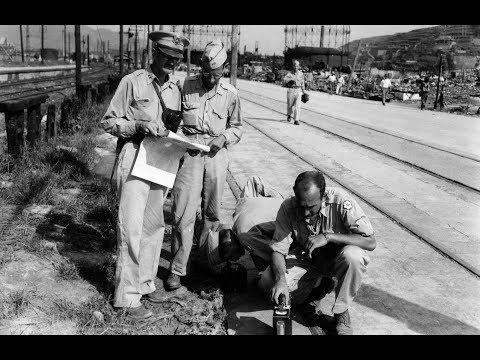
Jim Walther, director of the National Museum of Nuclear Science and History in Albuquerque, provides an overview of health physics and discusses the research Los Alamos National Laboratory has undertaken in the field.
Narrator: Jim Walther, director of the National Museum of Nuclear Science and History in Albuquerque, New Mexico, describes how Los Alamos has served as a pioneer in the field of health physics.
Jim Walther: Health physics is a form of medical and technical knowledge, where understanding the effects of radiation, the kinds of radiation, the types of materials that can cause radiation exposure, and what those things do to living tissue, how they have short and long-term effect.
They [health physicists] help to monitor the amount of radiation, whether it would be alpha, beta, gamma, how long it would be, how much. They calibrate the instruments that are used to detect and understand it, and they design the materials that protect us from that, the barriers as well as the administrative controls, like the kinds of signage and the kinds of warnings that would be around a radiological area.
What are the effects of long-term, chronic exposure to radiation, and what are the acute issues? When someone might have a burst of energy near them, what did that do? How did cell mutation take place, and what kinds of things were effective? What was the situation that occurred when you had radiation exposure?
Obviously, the dropping of the bombs in Japan, and then the military going in to learn about that and seeing for themselves how the Japanese people were affected. Those that lived from the very first blast and were exposed to radiation, many of those people were studied for many years. The [Los Alamos] laboratory became the place where those kinds of information were better understood, and it led to modern health physics.
Because radiation is a part of all of these kinds of work, in the medical community, but also in the weapons development community, in the power generation community. Every place that radiological materials are used, where they are in industrial settings, there is health physics.
There are people who measure and care for the amount of radiation and the experience that one has near it, and developed things like ALARA, As Low As Reasonably Achievable; elements of measurements; and things such as that. All of that started there.
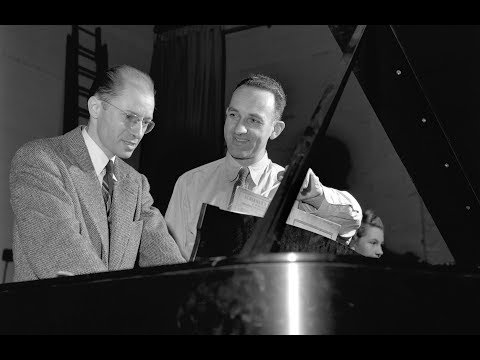
Dunell Cohn, son of Manhattan Project biochemist Waldo Cohn, and Manhattan Project chemical engineer Milton Levenson discuss how radioisotopes are used in medical research.
Narrator: Radioisotopes, the radioactive form of an element, were discovered to have tremendous medical value. Dunell Cohn, son of Manhattan Project biochemist Waldo Cohn, describes his father’s work to produce radioisotopes for medical use.
Dunell Cohn: He realized that you could use the reactor to produce radioisotopes in large quantities that could then be used for medical or biological research. He packaged and sent off the first radioisotopes produced in the reactor for that purpose of medical/biological research, and really started the radioisotope program at Oak Ridge.
Alvin Weinberg, the person who was the head of the Oak Ridge Laboratories for many years after the war, he decided that one of the major contributions that the whole Manhattan Project and Oak Ridge facility had made to the world was developing this program of making radioisotopes available.
Of course, they have had unlimited applications in research and in therapy. So it was an important contribution that came out of the war effort, even though it was not a direct objective.
Narrator: Manhattan Project veteran and chemical engineer Milton Levenson explains how the radioisotope technetium-99 is saving lives today.
Milton Levenson: There’s one isotope alone, tech-99, that over a million patients a year get treated with in the U.S. They estimate it reduces death of heart attacks in emergency rooms by 60 or 70 percent.
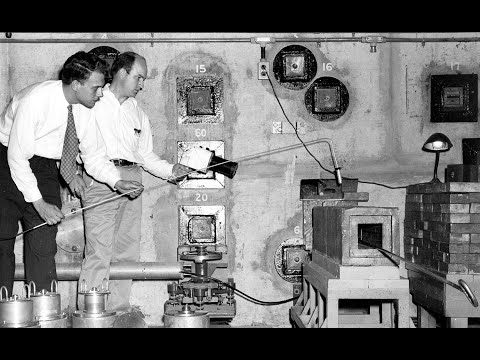
In 1946, the first shipment of reactor-produced radioisotopes for medical use was sent from Oak Ridge to the Barnard Free Skin and Cancer Hospital in St. Louis, Missouri. Medical isotopes are still produced at Oak Ridge National Laboratory today.
Narrator: Former manager of the Y-12 Plant Gordon Fee describes how the use of medical isotopes has grown at Oak Ridge since the 1940s.
Gordon Fee: Probably the number one innovation that has ever come out of Oak Ridge, Tennessee, is medical isotopes. In 1946, out of the Graphite Reactor at ORNL, the first isotope was shipped to a hospital in St. Louis, with the idea of using it as a possible treatment mechanism for disease.
But that first initial shipment blossomed into a multibillion-dollar business today. Today, one out of three of us in our lifetime will be subjected to some use of medical isotope, either for the treatment of cancer or the diagnosis of heart disease or cancer, or thyroid disease.
Many, many of the isotopes used throughout the years for that were produced on the same machine as the material for the bomb that was dropped on Hiroshima. And so, here you’ve got the terrible weapons use, and here’s the good for mankind, where these isotopes eventually become something that save lives and diagnose terrible diseases.
Narrator: Scientist Justin Baba explains that work continues at ORNL today.
Justin Baba: At Oak Ridge National Laboratory, you still have an isotope development program. And the purpose of that –– and a lot of people are not even aware of this –– is that the medical isotopes that are utilized for procedures today. The base material comes from the national labs. They’re developed at Oak Ridge and then the commercial vendors get those base materials, and they spin it in the cyclotrons and come up with all sort of radiopharmaceutical drugs that are utilized for everything from imaging to chemotherapy treatments.
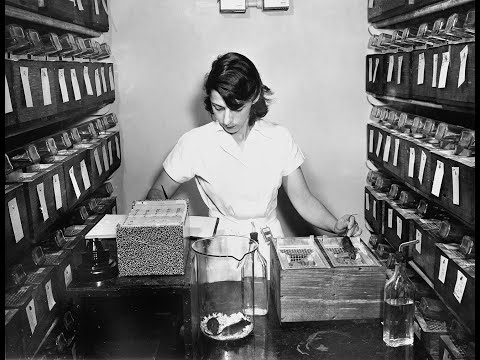
At Oak Ridge National Laboratory’s “Mouse House,” Bill and Liane Russell, along with a team of researchers, conducted groundbreaking studies on the effects of radiation.
Narrator: Bill and Liane Russell used Oak Ridge National Laboratory’s “Mouse House” to examine radiation’s relations to mutations. Former Y-12 Plant manager Gordon Fee explains.
Gordon Fee: In the early years, beginning in the ‘50s and up until the ‘80s, there was a Biology Division at Oak Ridge National Laboratory. And at one time had the largest pure stream of mice for experiments in the world. 250,000 mice was the peak. And there, started out very small, in the hundreds and then the thousands, and two very talented biology doctorates–a husband and wife team, Bill and Liane Russell–used those mice for years to study the intricacies of the human body.
Narrator: Liane Russell talks about experiments that led to guidelines to protecting women during pregnancy.
Liane Russell: I guess, the main mission of the program—to start with at least—was to determine radiation-induced mutation rates.
I found that there were critical periods in the [embryonic] development. At certain stages of development, you would get certain very specific types of effects.
When we tried to equate the time range in mouse development to human development, by picking, say, the same stage in humans where you get, I don’t know, the heart developed in a human and the heart developed in a mouse. Plotting all those things, it turned out that it’s the first seven weeks in humans—instead of fifteen days in a mouse—that was critical for major aberrations, abnormalities.
And so, anyhow, we developed this recommendation, because at that time, there was still a lot of medical irradiation used–X-rays–just for diagnostic purposes. And so, we recommended that–if it wasn’t necessary for survival, actually–if you could schedule irradiation, it should be restricted to the first two weeks following a menstrual period.
That became known as the—some people call it a ten-day rule; some call it a fourteen-day rule. Anyhow, it went into the medical literature, and it’s still in use now. The problem is most women don’t know they’re pregnant, so just being asked “might you be pregnant?” isn’t going to help. That’s why we set a specific time range.
Narrator: Bill and Liane Russell made many discoveries in biology and genetics. One of the most important: determining that in humans, many mammals, and some other species, the Y chromosome is what determines sex at birth. People with two X chromosomes tend to develop female characteristics; and one Y and one X, male characteristics.
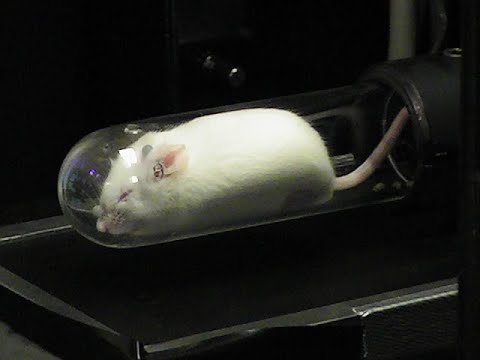
Medical researchers at ORNL today develop technologies that can be used to analyze brain functions and detect cancer, Parkinson’s, and other diseases.
Narrator: Oak Ridge National Laboratory scientist Justin Baba recalls his work on a project called “awake imaging,” which could be used one day to diagnose diseases like cancer, Parkinson’s, and Alzheimer’s.
Justin Baba: The awake imaging project’s goal was to image the brain. To do functional imaging of the brain, which means to look at brain function, to help be able to pick up stuff like Parkinson’s, Alzheimer’s and also neuroblastomas, which are really hard to diagnose. But to really do it non-invasively. So there was a team of us, including Oak Ridge National Laboratory, Thomas Jefferson National Accelerator Laboratory and Johns Hopkins University. And we actually developed a scanner that can image — and, we did the difficult thing, we did it in animals, in mice. So, we could image and track a mouse –– without any anesthesia, without any restraints, moving around –– and could image the brain and could literally pick up brain function.
So, that technology really needs to make it to the clinic. It will have a tremendous impact on brain diseases and related pathologies.
Narrator: TJ Paulus describes his work on another technology called positron annihilation.
TJ Paulus: Here, you have a source that would emit a gamma ray at one energy and a positron or two positrons. The positrons would meet an electron and annihilate. They would convert completely from a positive ion into a negative ion, which is an electron, and give off two gamma rays that are 180 degrees apart. Take two detectors and move them around, you can see which way things are going.
Positron annihilation is used in medical imaging. CAT scans, the gamma rays are coming out, and you actually go back and you can map the internal organ that you’re looking at where you have a cancer. And the positron will become more likely to land there or some other place that would give them, the medical person, a view of what you are down inside. So, it’s lots of applications, not just pure science.
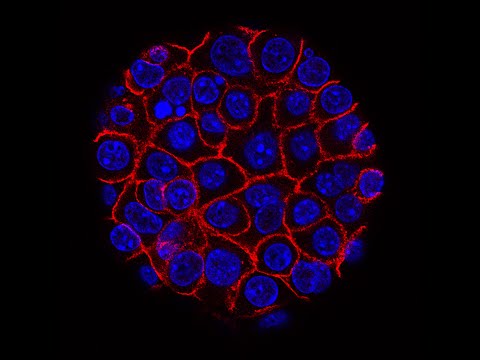
Julie Ezold discusses the use of alpha particles to attack cancerous tumors, in a process called targeted alpha therapy.
Narrator: Alpha particles are the heaviest form of ionizing radiation and they can be used to target and eradicate cancerous tumors. Scientist Julie Ezold explains.
Julie Ezold: There’s other work that Oak Ridge National Lab is doing with developing isotopes. A couple of them are what we call targeted alpha therapy. So, the isotopes, when they go through their half-life, emit those alpha particles, like the americium. Alpha particle is just like a helium nucleus—two neutrons and two protons. So, relatively speaking, we think it’s a big thing, and it packs a wallop. It has a lot of energy in it.
And the idea is if you could attach the isotope to a protein or an enzyme that would go to the cancer tumor—go through and attach itself—it would literally just start bombing that tumor from the outside and work its way in, with minimal damage to anything around it. So, I equate it to a smart bomb. It’s directly going to that tumor and it’s attacking the tumor and making it smaller and smaller.
And the initial results from the trials are absolutely amazing. One to two treatments, and the tumors are eradicated. So, it’s just that area of opportunity is boundless. I’m really hoping to see leaps and bounds in that area.