Atomic Science
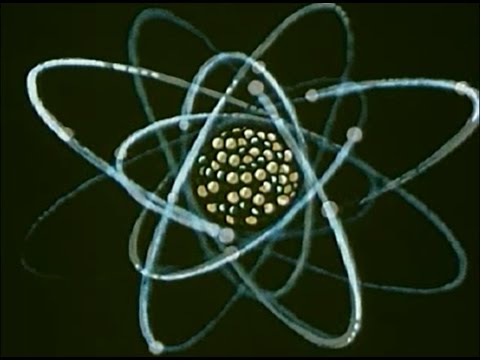
This animated short film, produced in the 1950s by John Sutherland Productions for General Electric, explains what an atom is and provides an introduction to nuclear energy.
Narrator: The Atomic Age was born. There is no denying that since that moment, the shadow of the atom bomb has been across all our lives. All men of goodwill earnestly hope that a realistic control of atomic weapons can and will be achieved. Meanwhile, good sense requires that all of us prepare for any eventuality. But wisdom demands, too, that we take time to understand this force. Because, here, in fact, is the answer to a dream as old as man himself, a giant of limitless power at man’s command.
And where was it science found that giant? In the atom, a particle so infinitely small that it takes over 100 billion billion atoms to make up the head of a pin. Just as other millions and quadrillions of atoms are the tiny building blocks which make up everything in the world: ships and shoes and sealing wax and cabbages and kings. Although no one has ever seen an atom, scientists have learned a great deal about how they behave, and there are widely accepted theories as to what they are like.
Let us start by meeting a leading authority on the subject, Dr. Atom. Now, observing the professor himself, we can see that his structure resembles, in many ways, something almost as vast as the atom is small: the solar system. And there are certain similarities. His is the center with electrons in surrounding orbits, but whereas the planets movement [inaudible], electrons is slightly different. There are other differences, too. Hey, hold it! Thank you.
Now, the solar system [inaudible] is electrical. The electrons, which are negative, are attracted by the protons, which are positive, and vice versa. But here in the nucleus are other particles with no electrical charge called neutrons, very important characters too, as we shall see. And equally important when it comes to atomic energy is what scientists call the atom’s binding force. It’s a kind of cosmic glue holding the nucleus together.
This then is a single atom, but certainly not all atoms are alike. There are in nature more than 90 basic elements, which is science’s term for families of atoms. To scientists, the atoms of the individual atom families, or elements, are identified by number, that is, the number of protons or positive charges in their nucleus. And they vary all the way from hydrogen, which has just one proton, to oxygen with eight protons, to gold—he’s rich with 79—finally on to the heaviest of all natural elements, uranium with 92 protons.
Now, within each element, or family of atoms, there can be different members, each one having the same number of protons, but differing in the number of neutrons. The total of an atom’s protons and neutrons is its atomic weight. Thus, in natural uranium, we have U-234, U-235, and U-238. These different members of the same element or atom family, science calls isotopes. Some elements, tin for instance, have a great many isotopes. Others, like aluminum, are lone wolves with just one.
Now, most atoms of most elements are content with their lot in life. We speak of them as being stable. But others are busy day and night being what science calls radioactive, like radium, throwing off powerful rays along with some of its neutrons and protons, until it actually alters its own nuclear structure and changes to another family, and then to another, until it does become stable at last. This spontaneous changing of elements is called natural transmutation.
Its discovery gave men of science an idea. If an atom could change itself, why couldn’t man change an atom? Using as bullets the very particles which radium threw off, a noted British scientist bombarded nitrogen and converted it to oxygen. In terms of individual atoms, this is what happened. The radium nucleus threw off an alpha particle consisting of two protons and two neutrons. One of the protons was absorbed into the nitrogen nucleus, turning it to oxygen. This was artificial transmutation, man changing the elements. From that first experiment, others by the thousands followed as scientists devised ever more powerful particle accelerators, commonly called atom-smashers, to transmute more and more kinds of atoms, all scientifically important but hardly world-shaking.
Then in 1939, some scientists were experimenting with transmutation of uranium. What would happen, they wondered, if they fired a neutron at a uranium nucleus, already the heaviest in nature? Why not try? So they tried, and the result: nuclear fission. Instead of a minor change, the atom split in two, truly a discovery to change the world. For what had happened when the uranium split was a kind of double miracle of science.
Half of the miracle concerned that binding force we spoke of before, that kind of cosmic glue which holds the atom’s nucleus together. We still don’t know all about that binding force yet, but we do know it is equivalent to mass. Therefore, we may speak of it as having a kind of weight of its own. Now, the two atoms into which a uranium atom splits also have binding force, but for some reason it takes less of that glue to hold them together, and in the process of fission, a tiny fraction is left over. What happens to it? It explodes as energy, proving Einstein’s theory that mass and energy are really the same.
But we spoke of a double miracle. To understand the second one, let us slow down that fission a million or so times. A single particle starts the reaction, splitting the uranium atom. Here now is the release of energy as heat and blast. Here are powerful rays being given off, similar to X-rays. But here, here are free neutrons driven out with tremendous speed. And provided there is sufficient U-235 present, what science calls a critical mass, those neutrons bombard other uranium atoms, causing them to split and split still others. The result: a chain reaction, over a million, billion, billion atoms exploding within two seconds. And the force? It would take Yankee Stadium full of dynamite to equal the energy released in the complete fission of an amount of U-235 the size of a baseball.
With this discovery at the time the free world faced a war for survival, it was little wonder the first thought was a weapon. But how to obtain enough material for even a single bomb? Only a small fraction of natural uranium is the U-235 isotope which will fission in a chain reaction, and to separate enough U-235 quickly enough seemed all but impossible. But the impossible became reality as industry, labor, science, and the military combined their efforts to build Oak Ridge, where enough U-235 was separated to build the first atomic bomb.
At Hanford, Washington, another impossible project proved possible when a huge plant was built for the mass production of the artificial element plutonium. This process involves what may be called the furnace of atomic energy, the reactor pile.
Here is a structure or pile of graphite blocks. In the reactor are placed rods of natural uranium containing both U-235 and U-238. As U-235 begins to fission, the graphite slows down the free neutrons and some of them hit other U-235 atoms, keeping the chain reaction going. But others of those slowed-down neutrons hit U-238 atoms, and here’s what happens. Remember, we said that U-238 would not support a chain reaction. However, it will capture neutrons from U-235 fission and start a process which converts the U-238, first to neptunium, then to plutonium. And plutonium will fission in chain reaction. Thus, the reactor itself is a source of atomic fuel.
Besides producing plutonium, the nuclear reactor makes possible two very important peacetime uses of atomic energy. Remember that the chain reaction process in the reactor creates tremendous heat, which scientists have learned how to control. Thus, a reactor may be substituted in many industrial applications, where heat is now provided by coal or petroleum.
But such uses in the foreseeable future are limited. For one thing, a reactor pile must be shielded to protect the workers around it from dangerous radiation, and this shielding adds tremendous weight. However, an atomic energy power plant has already proved feasible. The future supplying of electric power to entire cities is far from impossible, while nuclear power in locomotives, submarines, ships, and even very large airplanes may all but revolutionize future transportation on land, sea and air.
But perhaps the most valuable by-product of the nation’s reactor piles is radioactive isotopes. Research has revealed that many elements not naturally radioactive became so when placed in a nuclear reactor. And these isotopes, working as tracers with such measuring devices [0:12:00] as a Geiger counter, became invisible detectives, aiding the cause of science in many different fields.
In agriculture, isotopes are now used to test such things as the effect of fertilizers on plant growth and the proper timing for their use, helping to assure bigger and better yields from tomorrow’s farms. In industry, isotopes have found literally hundreds of new uses, such as the automatic thickness control of sheet aluminum, saving hundreds of man hours of labor and assuring accuracy never before possible.
In the fields of medicine and biochemistry, isotopes are performing near miracles of diagnosis and discovery. With radioactive sodium, doctors are solving more of the seeming mysteries of heart disease and circulatory disturbances. Radioactive phosphorous has been used to locate tumors in the brain and greatly simplify operations for their removal. Iodine-131 finds one of many uses in revealing conditions of the thyroid. And there are many more.
New ways of using isotopes are being discovered constantly through the tireless work of modern pioneers in such fields as chemistry, metallurgy, medicine, and biology. Truly the superpower which man has released from within the atom’s heart is not one, but many giants. One is the warrior, the destroyer. Another is the engineer seeking to provide vast quantities of energy to run the world’s machines. Another is the farmer, helping to better feed tomorrow’s world. Still another is the healer, helping to diagnose and cure the sick. And the last is the research worker, working on in the fields of pure science to reveal more of the mysteries of the universe.
But all are within man’s power, subject to his command. On man’s wisdom, on his firmness in the use of that power, depends now the future of his children and his children’s children in the new world of the Atomic Age.
MIT History Professor David Kaiser discusses the discovery of nuclear fission in 1938 and its immediate impact on countries around the world.
Narrator: Just before the outbreak of World War II came the discovery of nuclear fission and the splitting of the uranium atom in 1938. In a flash, Germany, Britain, France, Italy, Japan and the United States entered the race to build an atomic bomb.
David Kaiser: It’s sort of amazing in hindsight how quickly this whole prospect of nuclear weapons seemed to unfold. Accidental discovery of nuclear fission happened in a German laboratory near Berlin in very late 1938. Within just a few months, Europe had descended into war. This was right as war was about to break out where the notion of releasing energy via slitting a nucleus was first sort of hashed out.
News of that spread very accidentally away from the continent and reached the United States partly because young researchers who would work with Niels Bohr in Copenhagen swore him to secrecy. He was scheduled to take a steamer, a boat ride from Europe to the United States. He arrived early in 1939. He couldn’t help but blab to everyone he saw.
John Wheeler: In January 1939, Bohr came to Princeton on a visit so we had a chance to resume our collaboration. That was the middle of January when I went down to meet him here at the pier in New York. Of course, he had been told by [Otto] Frisch. Frisch and [Lise] Meitner had not wanted to tell Bohr until he got on the boat because they knew that he would be unable to keep the secret. He had to talk about a problem like this. He would have to talk with somebody. So here, all this pent up thought of his from the trip on the boat was discharged on me when I was there at the pier.
David Kaiser: Immediately, in Germany, in Britain, in the United States, in Japan as we now know, and in the Soviet Union, everyone realized there are weapons to be made here. And, this was not just another ho-hum scientific discovery. Everyone knew that this would have worldly implications within days and weeks.
We now know that every major power started its own nuclear weapons project within weeks of each other. Many of them stalled out. Many of them made very little progress. Others, like the Manhattan Project, ultimately succeeded in building these real weapons. It’s an interesting thought experiment. What if nuclear fission had been discovered either five years earlier or five years later? Let’s say it was discovered ho-hum you know, in 1933. The world was still a dangerous place. Dramatic developments in Central Europe with the rise of the Nazis, but would that have been, “Drop everything and work on this?” It’s not so clear. Certainly, if it was discovered five years later, you know, who knows what world we would be living in today.