Exploring the Reactor
The accumulators were large tanks filled with rocks from the Columbia River that shut the reactor down in an emergency.
Narrator: The B Reactor had several redundant safety systems in place. During everyday operations, nine horizontal control rods were electrically powered and manipulated by reactor operators to keep the rate of the reaction at optimal levels. To ensure that the control rods could still be inserted if electrical power were lost, engineers developed an ingenious system based on gravity. The system used what were called accumulators, which were really just large tanks filled with rocks from the Columbia River. The weight of the rocks held down stores of hydraulic oil under enormous pressure. During a power outage or other disruptive event, valves would be opened and the weight of the rock would force the oil through the valves, pushing the control rods into the reactor at a rate of 30 inches per second. The control rods could then absorb enough neutrons to shut down the reactor in all but the most extreme situations.
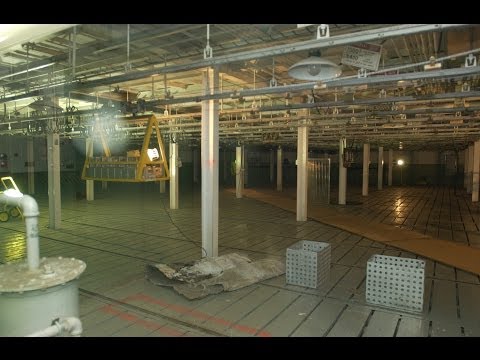
Irradiated fuel rods were pushed out of the back of the reactor into a deep pool of water. Hanford engineers Bob Egge, Paul Vinther, and Steve Buckingham discuss this and the process of cooling off the fuel.
Narrator: When the uranium fuel was sufficiently irradiated, the rod was pushed out the back of the reactor, down a shoot, and into a pool of water.
Paul Vinther: They shut the reactor down, cool it down, and they would take the caps off of the process tubes that were going to be charged-discharged. And then the people on the front face would then hook up the charging machine, and they would push out a few that had been in there.
Bob Egge: The worst thing in the world that you could do was push out a fuel element and it hung up someplace on the discharge side. It was out of water. It didn’t have any cooling. It was a pyrophoric material – if you get air on it, it will flash on you. It will burn itself right in the air.
Narrator: After being pushed from the process tubes, the uranium fuel would land in a shoot and slide down into the storage basin.
Paul Vinther: They had long tongs and they had a bucket, and they would put the fuel into that bucket. And they had a particular pattern they put it in, they knew how many levels of fuel elements would be in that particular bucket.
Bob Egge: You weighed a few baskets before you started, you weighed a few buckets after you had them fully loaded. Now you knew exactly how many fuel elements you had.
Steve Buckingham: Now here in the storage basin, the fuel sat for usually about 90 days to allow the short-lived fission products to decay away.
Bob Egge: The reason why you want to store them? Short-lived isotopes. What isotopes are really important? Iodine-131, the thyroid-seeking isotope. It collects in your thyroid. What happens when your thyroid gets bombarded with 131? It kills it.
Narrator: While the radioactivity from the fuel rods was very dangerous, the 20 feet of water in the storage basin shielded workers from the radiation.
Paul Vinther: There were not any special requirements for radiation protection. When the fuel first came out, you could take a look at it and it was glowing. You could see the hot fuel areas slowly cooling it out.
Steve Buckingham: The material that is discharged from the reactor, even after it decays for 90 days, is still extremely radioactive. They put it in these cask cars to take it up to the separations area. And between the cask cars and the locomotive, they would usually put in four or five blank railroad cars to separate the locomotive engineer from the cask car. And they would usually move the cask car at night, because they had to barricade the roadways because anybody waiting for the train to pass would get a pretty healthy dose of radiation.
Narrator: Handling irradiated fuel once it left the B Reactor required and received extreme caution.
This vignette follows the fuel slugs after they have been irradiated inside the B Reactor.
Narrator: After the fuel slugs had been irradiated inside the B Reactor, they were discharged out the back into a 20-foot deep pool of water called the storage basin. Men used 25-foot long tongs to grab slugs from the bottom of the pool and place them in steel buckets. The fuel slugs remained in the storage pool for up to 90 days, time enough for some of the radioactivity from the short-lived fission products to decay.
Next, the buckets of slugs were placed inside sealed, lead-lined railroad cask cars that were also filled with water. A locomotive would transport the cask cars to the massive, 800-foot-long chemical separation building called the T Plant about ten miles away.
Physicist Eugene Wigner convinced others that water was the best option to cool the reactor. Accordingly, the Columbia River water entered the reactor through the valve pit and then through pipes to cool the core of the reactor.
Narrator: Although water may seem like an obvious way to cool the reactor, it was not a given in the design process. After the successful demonstration of the Chicago Pile in December of 1942, scientists considered helium to be the best option for a cooling system, followed by heavy water, bismuth, and water was the fourth choice. The advantage of helium was that it would not absorb any neutrons, ensuring that the chain reaction would continue. However, the helium would have to be blown through the reactor at high pressure, and it would be difficult to filter out any contamination.
It was Eugene Wigner, a Hungarian émigré, who convinced the other scientists and engineers that water was the most practical solution. He proposed a design where bratwurst-sized slugs of uranium, sealed inside thin aluminum cans, would be loaded into aluminum tubes. Those would run through large graphite piles as water flowed around the slugs inside the tubes to cool them. This became the design of the world’s first plutonium production reactor, the B Reactor.
There were many engineering challenges to solve before this plan could be put into effect. For example, great care had to be taken to seal the slugs completely, in a process known as canning. Uranium is very reactive with water. If it comes into contact with cooling water, it rapidly oxidizes and creates a much more voluminous compound and blocks the flow of the coolant. And so a single ruptured or improperly canned fuel element could force the shutdown of the entire reactor.
Another issue was what to do with the cooling water. The solution for the B Reactor was to collect it in a basin at the back of the reactor, where it was stored for several hours before flowing back into the river. It was initially believed that this cooling water would not adversely affect the Columbia. Only later did scientists find that many of the radioactive contaminants were present hundreds of miles downstream.
To cool the B Reactor, an enormous amount of water was needed. In just five minutes, enough water flowed through the B Reactor’s process tubes to fill an Olympic-sized swimming pool.
Narrator: When General Groves and Colonel Matthias of the Army Corps of Engineers were deciding where to build this reactor, they considered several criteria. They were looking for a remote area, with abundant nearby sources of electric power and water. They looked at sites in Nevada and elsewhere, but one of the key factors in the decision to build the B Reactor here at Hanford was the newly completed Grand Coulee Dam, a major hydroelectric power source. The Columbia River flowed through 50 miles of the reservation, ensuring adequate water for cooling the reactors. Each reactor would be serviced by a water treatment plant large enough to supply the city of Baltimore.
The B Reactor had 27,000 gallons of water flowing though it every minute. The water took about a second from the time it entered the process tube until it was discharged out of the back. That was about 34 feet per second. During its brief stay in the reactor, the water’s job was to carry away heat, 80 million BTUs per gram of plutonium. That is the equivalent of the energy produced by 640 gallons of gasoline. And that’s why they needed so much water for cooling. The system was designed to take 27,000 gallons of water per minute through the reactor when it was operating at 250 megawatts, and then 72,000 gallons per minute when the power was increased to 2,090 megawatts in 1964. That meant that in just 5 minutes, enough water flowed through these process tubes to fill an Olympic-sized swimming pool.
The Fan Room pulled air out of the building, which was important to minimize any radioactive risks to workers, and dispersed widely through the stack.
Narrator: This is the Fan Room. The exhaust system here is known as a single pass air system, and that means the air is pulled out of the building rather than being recirculated. There are two electrical fans, and two steam fans for backup in case of power failure. Each one of those fans has the capacity to exhaust the building at a rate of 50,000 cubic feet per minute.
Exhausting the air out of the building was extremely important, due to the danger of radioactive contamination. Even though there was no real precedent for the kind of work that was being done at Hanford, and no one was exactly sure of the effect the radiation would have on the surrounding area, everything that could be done was done by the scientists to minimize the potential damage.
The first priority was the safety of the workers, so huge ducts connect every room in the reactor to the vents. That ensured that all of the air in the reactor could be evacuated very quickly. The second priority was to minimize the environmental and physical damage caused by the evacuated air. The huge smoke stack that you see outside of the building was designed to be so high that it would disperse any radioactivity into the winds, rather than having it fall back down on the reactor.
Hanford scientists and engineers redesigned the exhaust system to be more effective and reduce the risk of radiation spreading to nearby towns.
Narrator: When the B Reactor was first being planned, it was thought that expelling contaminated air through the stack would allow it to disperse into the atmosphere, diluting any radiation to such a degree that it would not be a danger. Unfortunately, this proved not to be the case. Instead, the meteorological and geological conditions at Hanford amplified the potential for so-called “sky radiation.”
Crawford Greenewalt was technical director of the Hanford project for the DuPont Company. In an early report, he explained that because the site was in a valley with shifting wind patterns, air emissions from the reactors might lead to a concentration of toxic elements where the valley ended in the bluffs. Equally problematic, contaminated air released from the reactor could drift toward Pasco. As a result, DuPont initiated studies of wind patterns, and determined that the danger was not as high as some feared.
Physicist Arthur Holly Compton wrote a report to help determine the height and placement of the stacks. And in August 1943, fans were added to increase the exhaust capability even further. Because of the top-secret nature of the project, residents of nearby areas had no idea of the possible threat to their safety, and many were understandably frustrated to learn what had been going on without their knowledge. It was not an easy task to balance concern for the workers and for the public, the need for complete security, and the goal to complete the project as quickly as possible.