Reactor Visit Preview
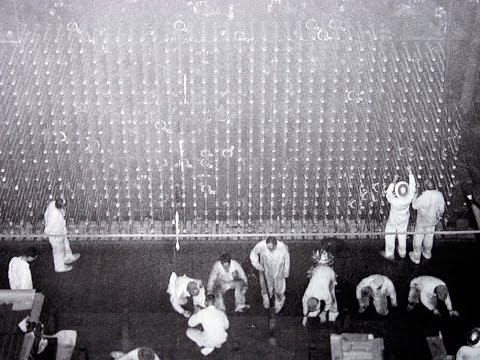
Hank Kosmata, an engineer and member of the B Reactor Museum Association, explains what you will see when you visit the B Reactor, and how the reactor operated.
Hank Kosmata: In back of me is the front face of B Reactor. It looks exactly like it did when this reactor went into operation in September of 1944. That’s a part of the mystery of B Reactor, because when you look at the reactor when it’s operating, nothing is moving. The only sound you hear is the sound of water running through the pipes. So there’s no way you can look at the reactor and get any idea of what makes it work.
There are two primary materials that actually are needed to make this reactor work: uranium in the form of metal rods—natural uranium—and graphite. Now, why did those two materials make it operate? There are three primary reactions that occur in this reactor. We would like to demonstrate to you how these reactions combine to provide the plutonium that this reactor produces.
There are three primary reactions that occur in this reactor: a fission reaction, a chain reaction, and a transmutation reaction that produces plutonium. We’ll start with the fission reaction. Early experiments determined that if you would slow down a neutron by bouncing it off of light atoms, you could produce a slow neutron, and a slow neutron would be accepted by the uranium-235 isotope. Uranium-235 isotope is less than 1% of natural uranium, .7%. But it’s the magic atom.
When the U-235 atom accepts the slow neutron, three important things happen. It breaks into two smaller atoms. It always breaks into two atoms, not necessarily the same pair. These atoms are called “fission products.” The fission products are unstable, and they kick out two to three neutrons with each reaction. Now, the combined atomic weight of the two fission products is very slightly less than the atomic weight of uranium-235, which means that we have lost weight or mass. Einstein’s theory says if you lose mass, you gain energy, and that’s exactly what happens in the fission reaction. Energy is produced, in this case as heat and gamma rays, which are just like intense X-rays.
Now, if we move from the fission reaction to the chain reaction, the chain reaction is a result of a number of fission reactions. When the uranium-235 releases three neutrons, for example, they in turn bounce off of the carbon atoms in the reactor and are slowed down until they intercept other uranium-235 atoms, and those atoms release two to three other neutrons. And so three neutrons become nine neutrons, become 27 neutrons, and it all happens in a flash.
Speed and time are a little different in physics. A fast neutron travels at 45 million miles an hour. A slow neutron travels at 5,000 miles an hour. Speed is different in nuclear physics. Time is different. Physicists talk about time in tiny fractions of seconds, microseconds, which are like a millionth of a second, or nanoseconds, which are like a billionth of a second. So a lot of things happen very fast in the fission reaction and the resulting chain reaction.
Now, why do we want all of these neutrons? In order to make the plutonium, we need a flood of neutrons, because those neutrons, again, are slowed down by passing through the carbon atom. When they intercept the uranium-238 isotope, which is most of natural uranium, the uranium-238 accepts the neutron, but it does not fission. Instead, it becomes a new isotope, uranium-239.
The uranium-239 is not a happy isotope. It’s unstable. In its attempt to become stable, it kicks out an electron and it changes itself into a brand new, man-made atom called neptunium. Neptunium is also not a happy element. It kicks out an electron and changes itself, or transmutates itself, into the plutonium-239, which is what we’re after in the first place.
These reactions don’t occur immediately. Physicists have a term they call ”half-life.” The uranium-239, for example, changes over a period of 23 minutes—half of it changes into neptunium. The neptunium half-life is 2.3 days. Half-life means half of the uranium-239 changes to neptunium in 23 minutes, and then in the next 23 minutes half of the remaining uranium-239 changes. In a matter of a few minutes, all the uranium-239 is changed to neptunium. In a matter of a few days, all the neptunium is changed to plutonium.
Plutonium is a very stable element. It sticks around for millions of years. However, plutonium has the unique characteristics that it too, under the right conditions, will fission. It fissions just like the uranium-235 atom, so it can be used in atomic weapons. So plutonium, as a weapons material, is able to be separated from the uranium, because plutonium is different chemically than uranium. Plutonium was a source of most of the weapons that were produced in this country, and they were the source of the first atomic explosions.
Now let’s learn a little bit more about the chain reaction. The first controlled chain reaction in the world occurred in Chicago. Once Enrico Fermi and Leo Szilard realized that uranium-235 fission releases two to three neutrons, they started working on a demonstration of a chain reaction. They used the two basic materials, uranium and graphite. They used graphite bars about this dimension, but about 16 inches long. They drilled holes down into the top of these solid bars. About a fourth of the bars were drilled to accept uranium pellets. In those bars, the spacing between the two pellet holes was about eight inches.
So they began with small experiments, which they called “piles.” They would stack solid uranium blocks, and then some of the blocks that contained uranium. They would inject neutrons from a chemical reaction into their pile, and then they would measure how many neutrons would be generated, how many new neutrons. They would then increase the size of the pile, and each time they increased the size of the pile, they realized they were getting more and more neutrons generated before it stopped. It stopped because in the small pile, neutrons escape out the sides and the top. They continued this process, and continued to measure and get more information on how this actually worked.
Now, in order to control the chain reaction that they hoped to get, they introduced one more material. Scientists had determined that there are some atoms that suck up neutrons just like a sponge sucks up water, and they call these “poisons,” they poison a reaction by absorbing neutrons. In their case, they used the material cadmium. They developed what they called “control rods,” which were just long sticks of wood with cadmium foil wrapped around the ends. As they built up these layers of graphite and uranium, they also left space for these control rods. Now their experiments would be to put the control rods into their pile, start some neutron generation and measure what was happening, pull the control rods out of the pile, repeat that. And again, they were learning more and more as they went along.
Until finally, in November of 1942, they had decided they now knew what size the total pile should be in order to get their goal of a chain reaction. So they began stacking these blocks in about a 25-foot diameter, with solid blocks on the bottom and then a row of blocks that some contained uranium and some were solid. They continued to build those up, pull their rods out, make their measurements, and continue building that pile, as they called it.
When they finally reached a level of about 20 feet, they were confident that they now could demonstrate a chain reaction. In fact, on December 2nd, 1942, when they pulled the control rods out from their pile, they found that the neutron generation was continuing, and it would continue until they injected the rods. In other words, they had finally demonstrated a chain reaction.
In the meantime, again at the University of Chicago, another group under Eugene Wigner was developing what would be the conceptual design for B Reactor. The difference between the production reactor and the demonstration pile at Chicago was that in order to generate the quantities of plutonium needed, you were going to have to have billions and billions of fissions, which meant an enormous amount of heat generated.
The Chicago pile never operated above 200 watts, like two lightbulbs. The reactor, B Reactor, was going to have to operate at a much, much higher power level, 250 million watts. That meant they had to develop a way to remove the heat from the fuel, because all the heat energy is generated in the fuel and if the heat is not removed, the uranium fuel will melt and ruin the reactor. They decided to use again the same basic components, graphite bars—just like the graphite bars in CP-1 [Chicago Pile-1], although these bars would not be four foot long. And they would drill holes through some of the bars longways, and they would provide a space for what they called the “process tube.”
The process tube would be aluminum. This is the section of the process tube that actually is in B Reactor. The process tube has little rails built into it when it’s formed. The fuel element, the uranium for the reactor, would be in the form of a cylinder about nine inches long, about an inch and a half in diameter, solid uranium, canned in aluminum. It’s canned in aluminum to protect the uranium from the hot water, because it would corrode otherwise. The solid fuel fits into this tube, rests on these little rails, and leaves a space around the fuel element for water to be introduced to remove the heat. The fuel element, or the process tube and the fuel element, fit into the hole in the graphite. This is the basic concept for this reactor.
The amazing thing about a reactor like this is, that these materials are all benign outside the reactor. This simulated fuel element is lead, about the same as uranium, although it would be a little heavier if it was uranium. But if it was uranium, I would handle it just like this. Natural uranium is benign. The operators who actually loaded the reactor picked them up out of a box with their hands and loaded them into the reactor.
The graphite: you’re familiar with graphite, because it’s the so-called lead in your lead pencils. This is a piece of graphite. You can write with it. When people discovered graphite originally, they thought it was lead. They called it black lead. The name stuck with it. But you have these benign substances: graphite; aluminum, just like the aluminum in your cookware; water, just water out of the Columbia River. When all these things are outside of the reactor, nothing happens. But when they’re in the right combination in the reactor, things go wild.
Now, we would like to introduce you to some of the models you’ll see when you come to visit our reactor. This model is made out of actual graphite that was left over from the construction of B Reactor. B Reactor Museum Association owns quite a few of these blocks, in fact, so we had our friends at Lockheed [Martin] make this model of the reactor heart. This is exactly what the reactor looks like if you went into the middle of the reactor.
There are two types of blocks used in this model and in the reactor. The so-called filler blocks are solid graphite running side-to-side, and there are filler blocks also running front-to-rear between the tube blocks. The tube blocks contain the process tubes. The process tubes contain the uranium slug. Of course, there’s a water annulus that allows the water to go through and cool the fuel elements.
In addition to these arrangements, in the top of the reactor there are 29 holes that drill all the way down through the reactor block, and those holes contain the safety rods. The safety rods hang above the reactor and in the event of certain emergency, safety rods drop into these vertical holes. In addition to that, there are slots for the control rods. The control rods in the reactor are aluminum tubes that contain a boron coating on the outside. They slide through these slots, nine of them coming from the left side of the reactor.
There are a number of features on this model that you’ll be able to see with pushbuttons that light up the specific highpoints. There are also a number of videos that are available in this model that will tell you how graphite is made, and a lot of other interesting things that you’ll obtain from this model when you visit this reactor.
Now, we’d like to go over and show you a physical model of the reactor. This reactor model was again made by our friends, Lockheed, using actual construction drawings and blueprints that were used to build B Reactor, but that show you a lot of things you can’t see just looking at the front face of the reactor.
On the side of the reactor, there are twin pipes called risers, 20-inch diameter pipes that deliver water to the reactor from the pump house, which is right outside of the reactor building. From the risers, small four-inch lines called “cross setters” supply water to each of the process tubes that run underneath the tube rows. The connection between the cross setter and the process tube is through a little curly pipe they call a “pigtail.” Now you have water coming into the riser through the cross setter into the reactor.
Now, I’d like to show you some other features. You can see from this cutaway how these 75,000 blocks were formed to make this total 36x36x28 block of graphite, how the process tubes go through the tube blocks.
Because when this reactor is operating, there’s an intense generation of gamma rays, which are like high-intensity X-rays, and an intense field of neutrons, both of which could be lethal to people outside. In order to protect the personnel, shields were manufactured of various materials. There’s cast-iron, and then there’s a composite of steel and Masonite. Masonite is a wood product, has hydrogen in it. The different materials do different things. The cast-iron and the steel stop the gamma rays, the hydrogen stops the neutrons.
Now, above the reactor, 29 safety rods were poised. They were dropped into the reactor by gravity in the event that the reactor lost coolant or that there was an earthquake or similar situations that might threaten the operation of the reactor. In such an event, these rods came crashing down into the reactor. That would stop the reactor immediately.
Normal control for the reactor is through nine control rods that come from the side. When the control rods are pulled out, the reactor starts to operate. When you want to slow down or stop the reactor, control rods, either all of them or some of them, are pushed into the reactor until finally in a normal shutdown, that’s how the reactor is stopped.
Now we’ll move over to the area map that will show not only the reactor, but all the other buildings that were necessary during the actual operation of this reactor. What you see behind you is a scale model of all the buildings and facilities that were needed during the operation of the B Reactor. The B Reactor building is an enormous building by itself. Over the top of the reactor, the ceiling goes up 100 feet. But if you try to find the reactor building, it’s a little difficult. It’s surrounded by buildings that are much bigger than it. I’ll give you a clue. The reactor building is way over to the right between the two high tanks. Again, all these buildings were made by 3-D printing. They’re exact from the actual construction drawings.
Everything else in this whole facility was there to provide a continuous, reliable source of water to the reactor. The reactor needed 30,000 gallons a minute when it was operating. Even when it was shut down, it required 5,000 gallons a minute. The water from the Columbia River comes from the river pump house, comes to a large reservoir and then moves into a filter plant, or a water treatment plant. This water treatment plant was way ahead of its times. Even though the Columbia River is very clean, any minerals in the water going into the reactor could become radioactive. They designed this very advanced water treatment plant to remove as much of the minerals as possible. The treatment plant is big enough to suit a large city. The DuPont engineers were from Wilmington, a city of 125,000, and they said this filtration plant would supply that whole city.
The water from the filtration plant goes to the facility, the biggest building on the site, which is the pump house in the Deaeration Facility. In the pump house, there are ten sets of pumps. Each set has two pumps. One supplied the power by electricity, the other by steam. If you lose one source of energy, another would take over. In addition to that, these pumps are large flywheels that will continue the operation of the pump for a minute or so even after you lose power. Again, all to make sure that there’s a reliable source of water at all times going to the reactor.
When the water goes to the operating reactor, it heats up. As it leaves the reactor, it goes to a facility back at the river, right before the river, called a retention basin. It flows to this basin for about four hours before it goes back into the river, so that any short-lived activity in the water will decay before its dumped back in the river. Then at that point, it goes back in the river.
Now, in addition to the normal operation, they had to plan for any kind of abnormal operations. For example, if you lost both sources of power, both electricity and steam, then you still had to have water to the shutdown reactor. That water would come from these high tanks. They’re 120 foot in the air and each of them contained 300,000 gallons, enough to supply the reactor for another two hours, and there’s also an export line coming from the other reactors. There were two other reactors ultimately went into operation at the same time as B, or a few months later, D Reactor and F Reactor. Each reactor site served the other reactors with this export line. Everything here is to make sure that this reactor always has a reliable source of water. All through its history, there was never any occasion when it didn’t have that reliable source of water.
When you realize that history was made with these dials, these controls, and these instruments, and that Enrico Fermi and his associates, scientists, and engineers were right here during the startup of this reactor; when you realize that atomic history was born in this facility right here, you can understand why BRMA is so excited to preserve this reactor and share it with you. Thanks for watching.
To return to the “Know Before You Go” tour, click the “Back to Tour” button at the top of this page.